Part 1: The Early Beginnings of Climate Science: Fourier & the Greenhouse Effect
The idea of a greenhouse effect has been around since Fourier. Indeed, the greenhouse effect is a good thing when kept in check as it keeps Earth warm enough to support life. The issue that we're facing now is that we're out of equilibrium - too much of a good thing. Then, learn how the observations of an amateur naturalist led to important scientific theories regarding Ice Ages and climate change. Finall
y, we discuss the early studies that connected carbon dioxide to climate and global temperature.
Ironically, one of the theories proposed to explain what had caused the Ice Ages to come and go would become the key argument supporting an opposite, and equally controversial, kind of climate change: global warming. In 1895 Svante Arrhenius proposed that the drop in temperatures that occurred during the Ice Age could have been produced by a drop in the concentration of atmospheric carbon dioxide compared to the then-current levels. He even proposed that industrial emissions could raise Earth’s temperature in coming centuries.
Despite his theory, most scientists didn’t believe that humans could affect climate until the 1960s, when evidence of human impacts on the natural world were becoming increasingly obvious. Once again, climate change became controversial, as the theory of global warming became one of the biggest scientific debates of the late twentieth century. The questions of the past generation took on a new urgency. Could climate change be a threat to humankind? And could our own actions increase the threat?
These questions have compelled scientists to scour the Earth for signs of past climate change. Their search has developed into an entire scientific field: paleoclimatology, the study of past climates. In determining what has triggered climate change in the past, scientists hope to learn how natural and human triggers might change our climate in the future.
Most scientists who study the past have fossils and artifacts that help them reconstruct history. But without thermometer readings, how can they know how cold it was when the ice from the last Ice Age began to retreat? Scientists who want to reconstruct past climates gather clues buried in the Earth in much the same way that an archeologist reveals past culture by looking at artifacts or a detective reconstructs a crime scene using multiple bits of evidence.
Climate leaves an imprint on the planet, in the chemical and physical structure of its oceans, life, and land. Some of these artifacts, known as climate proxies, reveal general climate patterns over the entire Earth, while other proxies reveal seasonal change in specific regions. By reading the signs of past climate, scientists reconstructed the history of Earth’s climate over hundreds of thousands—in some cases millions—of years. When combined with observations of Earth’s modern climate into computer models, paleoclimate data help scientists to predict future climate change.

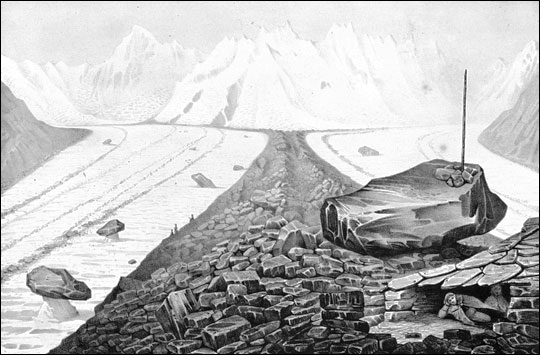
Ironically, one of the theories proposed to explain what had caused the Ice Ages to come and go would become the key argument supporting an opposite, and equally controversial, kind of climate change: global warming. In 1895 Svante Arrhenius proposed that the drop in temperatures that occurred during the Ice Age could have been produced by a drop in the concentration of atmospheric carbon dioxide compared to the then-current levels. He even proposed that industrial emissions could raise Earth’s temperature in coming centuries.
Despite his theory, most scientists didn’t believe that humans could affect climate until the 1960s, when evidence of human impacts on the natural world were becoming increasingly obvious. Once again, climate change became controversial, as the theory of global warming became one of the biggest scientific debates of the late twentieth century. The questions of the past generation took on a new urgency. Could climate change be a threat to humankind? And could our own actions increase the threat?
These questions have compelled scientists to scour the Earth for signs of past climate change. Their search has developed into an entire scientific field: paleoclimatology, the study of past climates. In determining what has triggered climate change in the past, scientists hope to learn how natural and human triggers might change our climate in the future.
Most scientists who study the past have fossils and artifacts that help them reconstruct history. But without thermometer readings, how can they know how cold it was when the ice from the last Ice Age began to retreat? Scientists who want to reconstruct past climates gather clues buried in the Earth in much the same way that an archeologist reveals past culture by looking at artifacts or a detective reconstructs a crime scene using multiple bits of evidence.
Climate leaves an imprint on the planet, in the chemical and physical structure of its oceans, life, and land. Some of these artifacts, known as climate proxies, reveal general climate patterns over the entire Earth, while other proxies reveal seasonal change in specific regions. By reading the signs of past climate, scientists reconstructed the history of Earth’s climate over hundreds of thousands—in some cases millions—of years. When combined with observations of Earth’s modern climate into computer models, paleoclimate data help scientists to predict future climate change.
![]() | |||||||||||||||||||||||||||||||||||||||||||||||||||||||
by Holli Riebeek· design by Robert Simmon· December 22, 2005
While cave rocks and ice cores provide a long-term, annual record of past climate (see “Written in the Earth” and “The Ice Core Record” in this series), some other climate proxies can offer a detailed record of seasonal temperature or rainfall changes. As they grow from season to season, coral reefs in the oceans and trees on the land both record small variations in the climate. These records can tell scientists about growing conditions in the oceans or on the land, but the record only stretches across the collective lifetimes of the organisms that have been preserved through the centuries. Thus, even though their records are more detailed, reefs and trees cannot provide records that are as long or continuous as ice or sediment core records.
|
Some features of this site are not compatible with your browser. Install Opera Mini to better experience this site.


This page contains archived content and is no longer being updated. At the time of publication, it represented the best available science.
![]() | |||
by Steve Graham • January 18, 2000![]() Ironically, Arrhenius’ education and training were not in climate research, but rather electrochemistry. His doctoral thesis on the chemical theory of electrolytes in 1884 was initially regarded as mediocre by his examination committee, but later was heralded as an important work regarding the theory of affinity. In 1891, Arrhenius was a founder and the first secretary of the Stockholm Physical Society, a group of scientists whose interests included geology, meteorology, and astronomy. His association with this society would later help stimulate his interests in cosmic physics—the physics of the Earth, sea, and atmosphere. In 1903, Arrhenius was awarded the Nobel Prize for Chemistry for his work on the electrolytic theory of dissociation. In the years following his international recognition, Arrhenius lectured throughout Europe and was elected to numerous scientific societies. next: Arrhenius' Carbon Dioxide Research | ![]() Svante Arrhenius Arrhenius' Carbon Dioxide Research Hot House Theory Links and References Images Top: A portrait of Svante Arrhenius. (By Hailey M. King) |
No comments:
Post a Comment